Action Potential: Probing the Brain Power of Pitt Neuroscience
Although it is a three-pound lightweight, the brain is the human body’s uncontested heavy hitter. Its 100 billion neurons control the seat of all knowledge, power the central nervous system, and cradle the soul of our individuality. We have mapped its lobes, nerves, and blood vessels; we have labeled its cells, divided its activities into motor, cognitive, and sensory; and sorted its matters according to color—gray, white, and substantia nigra (Latin for black stuff).
Despite these achievements, there are significant gaps in our understanding. But day by day, University of Pittsburgh neuroscience faculty work to unlock the brain’s remaining secrets, attracting considerable research support to the University, primarily from the National Institutes of Health (NIH).
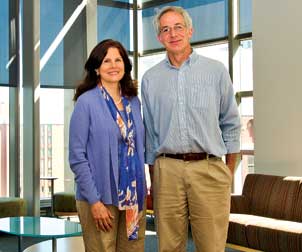
Throughout the past two decades or more, Pitt has built itself into a leader in brain research, focusing on its strengths in basic and clinical science. A spirit of collaboration is engendered in this work through the Center for Neuroscience, a University-wide center encompassing neuroscience on campus. The center’s diverse and multidisciplinary nature is reflected by the more than 30 different academic departments and centers in which more than 100 faculty conduct neuroscience research. Doctoral and postdoctoral trainees also play a major role in the center’s research activities, as do students in the highly successful undergraduate neuroscience major in the School of Arts and Sciences’ Department of Neuroscience.
Neuroscience research at the University runs from conducting basic cellular science to developing medications to building prostheses to replace damaged limbs. It includes work done through the Department of Psychiatry in the School of Medicine (and its clinical affiliate, Western Psychiatric Institute and Clinic [WPIC] of UPMC), for decades a national leader among NIH-funded psychiatry departments, which received about $90 million in total research funding in 2010. It also includes work through the Center for the Neural Basis of Cognition (CNBC), a joint venture of the University of Pittsburgh and Carnegie Mellon University that leverages the strengths of each to support a coordinated research and education program of international stature.
The following profiles in neuroscience research by prominent members of our faculty are just a sampling of the exceptional neuroscience enterprise here at the University of Pittsburgh.
Mapping Molecular Messengers
Susan G. Amara, the Thomas Detre Professor and chair of neurobiology and president of the prestigious Society for Neuroscience, studies the transport proteins responsible for the reuptake of neurotransmitter molecules released during chemical neurotransmission in the central nervous system. One family of transporters clears glutamate, a major excitatory transmitter in the brain that limits the temporal and spatial range over which glutamate molecules can signal. Because excessive amounts of glutamate can trigger neuronal cell death, glutamate transporters also protect the brain from the toxic actions of glutamate.
Brain cell death lies at the root of many central nervous system disorders, including ischemia, or stroke; amyotrophic lateral sclerosis (Lou Gehrig’s disease); Parkinson’s disease; and Alzheimers disease. Understanding cell-death mechanisms could help researchers to identify new drug targets and interventions, says Amara, a member of the National Academy of Sciences and a former Howard Hughes Medical Institute investigator. “We know that extracellular levels of glutamate rise in ischemia,” she says. “If we could learn how to enhance glutamate removal, we might be able to protect the brain from stroke damage.”
The brain’s most common neurotransmitter, glutamate accounts for some 90 percent of brain activity and is known to be involved in cognitive functions, learning, and memory. It is crucial for normal neuron function and survival. Yet system imbalance can lead to surplus glutamate accumulation—also known as excitotoxicity—and cell death.
“How can we prevent the brain from having too much of a good thing?” asks Amara. “By understanding glutamate transporter structure and mechanisms, we hope to develop molecules that increase the rate of different steps in the transport cycle, reduce ambient glutamate and, thus, limit the damage from excitotoxicity.”
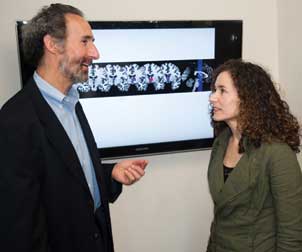
Among candidate molecules is a neuroprotective compound purified from the paralyzing venom of Parawixia bistriata, a social spider native to Brazil. The venom component selectively enhances glutamate transport, and Amara’s group is working with chemists to define the configuration of the active compound, given its potential as a basis for the development of new stroke drugs and treatments for other neurodegenerative disorders.
Amara also studies transporters for dopamine, norepinephrine, and serotonin—important neurotransmitters with key roles in regulating brain circuits involved in mood, reward, attention, and activity. Of particular interest are investigations into how certain drugs—including the psychostimulant drugs of abuse amphetamines and cocaine, attention deficit hyperactivity disorder (ADHD), medications like Ritalin, antidepressants like Celexa and Prozac, and other major classes of therapeutic and illicit drugs—affect the brain.
“These drugs have many more actions than we previously thought,” explains Amara. “We have always assumed that psychostimulants such as amphetamine and methamphetamine potentiate the actions of neurotransmitters by blocking reuptake, but we never realized that they also have their own specific receptor targets within the cell. Our new work shows that the drugs enter the cell cytoplasm to activate specific signaling pathways and alter the surface distribution of key regulatory molecules involved in neuronal communication.” These observations change the way we think about how the brain is altered by repeated drug use and why amphetamines have such a profound and distinct addictive potential.
Other University investigators parse the biochemistry of these cellular-level events for influences that reach beyond physiology to the heart of the human psyche.
Roots of Behavior
Daniel S. Shaw, professor and chair of psychology in the School of Arts and Sciences, has spent more than 20 years probing the foundations of antisocial behavior in children, looking for ways to successfully intervene before it’s too late. He leads longitudinal studies to follow children from disadvantaged, troubled families—one set of boys starting at 18 months of age who are now 20 years old, and another group of 10-year-old boys and girls who were initially enrolled at age 2.
“When kids got to age 10, 11, or 12, they were very tough to get out of that problem behavior, and they had burned their parents so many times,” he says. “At 10 and 12, you’re talking about kids starting to go underground and not just lie and steal, but hurt things. Some of our more precocious kids were using drugs and selling them.”
Rich collaborative opportunities have now added a facet of neuroscience to his behavioral research. “We have funding from the National Institute on Drug Abuse to look at the effects of genetics on brain function, the effects of environment, and the interactions between genetics and environment,” Shaw says, gesturing so excitedly he practically vibrates with anticipation. “This is my first entry into neuroscience.” For the project, he is working with Erika Forbes, assistant professor of psychiatry in the Pitt School of Medicine, and Ahmad Hariri, former director of Pitt’s Developmental Imaging Genetics Program, who is currently a Duke University neuroscientist.
“We have over 100 brain scans so far—unusual for this literature—but we have an incredible cohort,” says Shaw. “We hope to have a breakout paper when it’s all ready [in late 2011].”
Your Brain on Adolescence
For Beatriz (Bea) Luna, a professor of psychiatry in the School of Medicine, and of psychology in the School of Arts and Sciences, there is no question that maturity modifies brain function. Winner of a 2005 Presidential Early Career Award for Scientists and Engineers, Luna uses functional magnetic resonance and diffusion tensor neuroimaging technologies to study how brain mechanisms underlying cognitive skills develop during adolescence—a period often marked by a spontaneous combustion of risk-taking, misjudgments, crisis-level mood swings, and impulsivity.
“As a teenager, I remember thinking that something extremely special was going on and at the same time knowing it was temporary,” says Luna, who is also founding director of the Laboratory for Neurocognitive Development at WPIC. “I looked at my parents, and I knew they didn’t think in the same way that I did.”
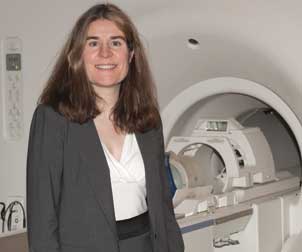
Connections between neurons—called synapses—multiply rapidly during the first two years of life, followed by a process to cull superfluous connections and strengthen those used most frequently. Scientists believe this process allows the brain to adapt to an individual’s particular environmental needs.
“Adolescence is not a disease,” she says with a smile. “It’s a necessary time of brain development, where young people should be encouraged to explore, gain experience and independence, and sculpt their future selves.”
Selected by the NIH to serve as a member of its Advisory Committee to the Director, Luna also has advised the U.S. Supreme Court on sentencing guidelines for adolescent offenders. “As we understand more and more about how the brain works,” she says, “we can try to understand breakdowns that may lead to conditions like ADHD, autism, and mental illnesses like depression and schizophrenia.”
A Matter of Mental Health
Molecular neurotransmitter pathways also intrigue David A. Lewis, UPMC Professor of Translational Neuroscience and chair of psychiatry, who investigates pathways’ relevance to the devastation of mental illness.
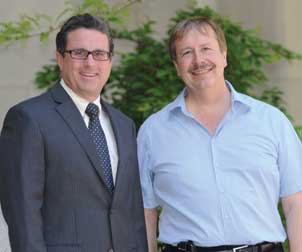
“Brain circuitry abnormalities appear to underlie schizophrenia,” says Lewis, who is also director of Pitt’s National Institute of Mental Health-funded Conte Center for the Neuroscience of Mental Disorders. “These abnormalities change with development and may generate different symptoms, yet are interconnected.”
A complex disorder, schizophrenia affects more than 1 percent of the population. Clinical symptoms typically become apparent in teenagers and young adults, and the disorder frequently results in a lifelong struggle against severe cognitive and social challenges. Symptoms range from disordered thoughts, delusions, and hallucinations to motivational problems and an emotional “absence” called flatness. There can also be difficulties in thinking, attention, and memory.
“That diversity of symptoms clearly suggests that multiple regions of the brain are involved and multiple circuits are disturbed,” says Lewis. “Our goal in devising new treatments is to reduce the causes and their negative consequences and to augment the brain’s own compensation mechanisms.”
Current medications primarily target delusions and hallucinations; there are few treatments available that help patients to manage disordered thinking, motivation, or memory. A troubling susceptibility among many with schizophrenia to fall prey to addictions—perhaps as a form of self-medication—also presents challenges.
Among Lewis’ recent publications are studies of the biochemical relationship between the neurotransmitter gamma-aminobutyric acid (GABA) and a key ingredient in marijuana that suggest a biological basis for population studies that connect marijuana use during adolescence with an increased risk of developing schizophrenia. In addition, the course of illness is worse for people with schizophrenia who use marijuana, he says.
Since GABA is known to be reduced in schizophrenia, these findings suggest possible new drug targets that could improve brain function in individuals with schizophrenia.
One of the most studied brain areas is the dorsolateral prefrontal cortex, which houses executive processes and working memory, says Lewis, defining working memory as the ability to remember information transiently and use it to guide behavior or thought.
“Now we are working to decipher the specific components—the molecules, cells, and synapses—and the larger circuits present in the dorsolateral prefrontal cortex that are behind this disturbance,” he adds.
Among these components, morphological evidence reveals that some neurons may even be smaller and have fewer dendritic protrusions called spines, which help to transmit electric signals to the neuron. Other neurons exhibit variations in gene product expression.
“There may not be a classic neuropathology of schizophrenia similar to the tangles and plaques characteristic of Alzheimer’s disease,” says Lewis. “But that doesn’t mean there aren’t changes in the brain that are, in fact, the basis of the illness.”
Neurotransmitters Gone Wild
Brain regions associated with schizophrenia include the hippocampus and prefrontal cortex. Drug use and environmental toxins are implicated in its development; there are also several candidate genes and possible roles for the neurotransmitters dopamine, glutamate, and serotonin. Rodent models of schizophrenia developed at Pitt point strongly to the hippocampus.
“The question is, ‘What’s wrong?” says Anthony A. Grace, Distinguished Professor of Neuroscience in the School of Arts and Sciences, who helped to develop this study. Among his observations: There is hyperactivity in the hippocampus because of altered GABA transmission that ultimately results in dopamine system overdrive.
“Normal GABA activity is essential for proper cortical structure functioning,” Grace notes. GABA loss, in turn, disrupts other key brain cell communications involving stimulus recognition and higher cognitive function, he adds.
Grace believes similar neuron and GABA losses in the prefrontal cortex also contribute to cognitive deficits associated with schizophrenia. In addition, other characteristics are exhibited that are consistent with human schizophrenia patients, including amplified startle reflex, altered executive function, and hyperresponsiveness to drugs of abuse like phencyclidine and amphetamine.
“We’re working with a chemist at the University of Wisconsin to design drugs specific to the alpha 5 GABA receptor in the hippocampus. These drugs are like Valium and other benzodiazepines in that they amplify GABA. These seem to reverse this physiological and behavioral response and could turn out to be a new antipsychotic,” says Grace.
Pittsburgh Institute for Neurodegenerative Diseases
Located in Pitt’s state-of-the-art Biomedical Science Tower 3, the Pittsburgh Institute for Neurodegenerative Diseases (PIND) brings together scientists who are working on degeneration from a number of perspectives. But investigators have one collaborative goal: to conduct successful translational research toward potential therapies for neurodegenerative diseases.
J. Timothy Greenamyre, UPMC Professor of Movement Disorders and vice chair of neurology and PIND director, studies the mechanisms that cause nerve cell death in disorders like Parkinson’s, Huntington’s, and Alzheimer’s diseases.
“What is it in Parkinson’s that makes dopamine neurons so vulnerable?” Greenamyre asks, listing mitochondrial abnormalities and systemic exposure to environmental toxins among likely suspects. “Odds are that genetics loads the gun, and something in the environment sets it off.”
Considered a world expert on mitochondria, the “power plants” of living cells, Greenamyre becomes particularly passionate about rotenone. A commonly used pesticide, rotenone until recently had support even among organic food producers and home gardeners since it is found naturally in a number of tropical plants. “We used to sprinkle it on our tomato plants,” Greenamyre says, grimacing.
Classified as moderately hazardous by the World Health Organization, rotenone targets mitochondria. In studies of a model of Parkinson’s disease, researchers found that rotenone “acts systemically, yet is specifically neurodegenerative,” says Greenamyre. Based on Greenamyre’s work in the laboratory, a recent epidemiological study has confirmed that human exposure to rotenone is a risk factor for the disease.
Other Greenamyre investigations involve reactive oxygen species and alpha-synuclein. “We know that alpha-synuclein is a major player,” Greenamyre says of the protein, which is a significant component of Lewy bodies—clumps of proteins that are a clinical hallmark of Parkinson’s. Alpha-synuclein is found throughout the central nervous system, but its function in the healthy brain is currently unknown.
Edward A. Burton, assistant professor of neurology and of microbiology and molecular genetics, is developing zebrafish models of progressive supranuclear palsy, a rare brain disorder that causes Parkinson’s-like disabilities yet does not respond to Parkinson’s medications. Developed through genetic modification, these fish overexpress a form of human neuronal Tau, a protein also associated with Alzheimer’s disease and dementia secondary to traumatic brain injury.
“We see age-related progressive neuropathology and motor difficulties in adult animals,” says Burton, who has also engineered Tau zebrafish for screening. Using these models, Burton and colleagues hope to identify therapeutic targets for the development of new candidate drugs. Already, he has been able to establish noninvasive evaluation methods that are sensitive to neural dysfunction and could be used to detect repair mechanisms.
Pittsburgh Compound B
Perhaps the most significant diagnostic advance in Alzheimer’s research today is Pittsburgh Compound B (PiB). Developed and tested by William E. Klunk, professor of psychiatry, and Chester A. Mathis, professor and director of Pitt’s PET Facility, PiB allows clinicians to see amyloid plaques in the living brain of individuals with Alzheimer’s and also in those who do not yet exhibit signs of the disease. This finding could lead to earlier diagnosis, illuminate disease progression, and uncover prevention strategies.
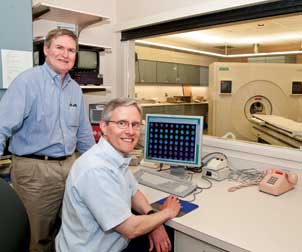
PiB imaging can also be used to differentiate Alzheimer’s from other forms of dementia and to directly measure the effects of antiamyloid therapies currently in development. For their work, Klunk and Mathis have earned major honors, including the Ronald and Nancy Reagan Research Institute Award for outstanding contributions to research, care, and advocacy for Alzheimer’s disease, and the Potamkin Prize, often called the “Nobel Prize” for neurology.
Viral Vectors Blaze the Trail
Peter Strick, professor of neurobiology, uses rabies and herpes simplex viruses to “envision” neural pathways related to movement.
With the viruses revealing chains of synaptically connected neurons, Strick has uncovered new evidence that the brain’s basal ganglia and cerebellum are linked to form an integrated functional network. The findings provide a neural basis for a cerebellar contribution to some of the disabling symptoms of basal ganglia disorders like Parkinson’s and dystonia and could point to new treatment approaches, Strick and colleagues reported in the May 2010 Proceedings of the National Academy of Sciences.
“I’ve always been interested in the nature of volition,” says Strick, also codirector of the Center for the Neural Basis of Cognition. “How is it that when I want to move my finger, it moves?’ What regions of the brain are involved in that?”
Strick investigates the essential connections between function and physiology, cognition and behavior. Using viral markers, Strick can work neuron to neuron, linking neurons like individual cars that together give purpose to a speeding train.
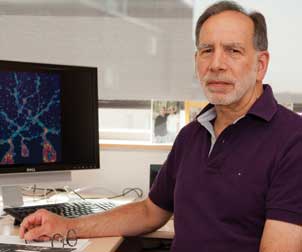
“We can work out the circuitry,” Strick says, listing potential investigations to further elucidate the connection between the basal ganglia and cerebellum. “There’s growing evidence, for example, of cerebellar involvement in addiction and fear response,” he continues, adding that knowing how such connections work could lead to new treatments not only for addictions, but also for post-traumatic stress disorder.
In previous work, Strick mapped a new area of the cerebral cortex that has evolved to enable humans and other higher primates to perform fine motor tasks. His findings show that the brain’s primary motor cortex has adjacent “old” and “new” regions. But unlike the older, less direct region more active in lower animals, this newer region directly controls spinal motor neurons to activate shoulder, elbow, and finger muscles, refining movements required for complex tasks like playing a musical instrument. In recognition of his seminal findings in neural tracing studies, Strick was elected a Fellow of the American Academy of Arts and Sciences in 2004.
Translational Science and Stroke
“There are so many diseases where neurons die—degenerative diseases like Huntington’s, traumatic brain injury, stroke,” Robert M. Friedlander, professor and chair of neurological surgery, says with a sigh. “Causes may differ, but many of the pathways mediating cell suicide are shared. As we better understand these pathways, we can be better prepared to develop novel therapies for these often devastating and untreatable diseases.”
Friedlander’s lab works with models of stroke, spinal cord injury, Huntington’s disease, multiple sclerosis, and ALS, testing drug libraries for potential new uses. Experiments have shown that the antibiotic minocycline appears to delay disease progression, along with the hormone melatonin and the antiglaucoma drug methazolamide. Clinical trials with minocycline are now under way.
The third-most common cause of death, stroke is the leading cause of disability in the United States. Triggered by blood-flow disruptions, a severe lack of oxygen catapults the immediately involved nerve cells into metabolic collapse and necrosis. Neurons further downstream suffer low-oxygen stress, activating an endogenous cell-death pathway and leading to cell self-destruction (apoptosis.)
Considering the devastation caused each year by stroke—the American Heart Association estimates that someone suffers a stroke every 40 seconds, on average, and dies every four minutes—it is vital to seek more and better treatments.
“Often it is difficult to stand out in many areas, but at Pitt, neuroscience has enough breadth to truly excel,” Friedlander says. “We also bring that to clinical practice.”
Because sometimes you just have to resort to the scalpel. At Pitt, intracranial aneurysms are evaluated by a multidisciplinary team of neurosurgeons and neurologists, including experts in microvascular and endovascular surgery. Some brain and spinal cord tumors can be treated endoscopically—literally through the nose, with microsurgery, or radiosurgery. Subspecialists are additionally trained in complex and minimally invasive techniques for spine, skull base, neuro-oncologic, pediatric, chronic pain, and stereotactic surgery, as well as acute stroke intervention.
Building a Brain-Computer Connection
One of the bravest of new worlds, though, may be found in the laboratory of Andrew B. Schwartz, professor of neurobiology. Working with Michael L. Boninger, professor and chair of physical medicine and rehabilitation, Schwartz and colleagues are getting nearly $7 million over the next three years from the NIH and the Defense Advanced Research Projects Agency to test a multifunctional prosthetic limb with spinal cord injury patients. The prostheses, mounted on wheelchairs, will be controlled by the patients’ brains through implanted electrodes connected to a computer.
“There’s a lot of work that we have to do, but the good thing is that we’re pretty sure it’s going to work,” says Schwartz, whose confidence is perhaps understandable given a landmark 2008 Nature article he authored on the subject.
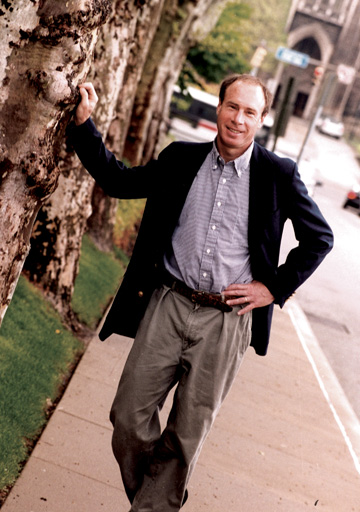
The newest trial will encompass three patients with different connectors. The first patient will have two, the second patient will have one, and the third patient will test a wireless telemetry version of the interface. In a companion project, additional patients will test an electrocorticography (ECoG) version of the interface using a grid placed on the surface of the motor cortex for up to 29 days. Neural activity captured by ECoG will be translated by a computer processor to allow the patient to control computer cursors, virtual hands, computer games, and assistive devices such as a prosthetic hand or a wheelchair.
“We want the patient to use the robotic arm to reach out, grab objects, and interact,” says Schwartz, explaining that the sensory cortex of one patient’s brain will also be stimulated by an implanted electrode array, allowing him or her to “feel what the robot hand is doing.”
These examples only hint at an intensity of neuroscience expertise that the University of Pittsburgh shares with few institutions. Arthur S. Levine, senior vice chancellor for the health sciences and dean of the School of Medicine, says he can envision the future development of a Neuroscience Research Institute similar to the current University of Pittsburgh Cancer Institute. Such a center could support, recruit, and focus the collaborative brain power of talented faculty to further reveal the organ that Luna calls “this wonderful machinery that holds the secret of who we are.”
Other Stories From This Issue
On the Freedom Road
Follow a group of Pitt students on the Returning to the Roots of Civil Rights bus tour, a nine-day, 2,300-mile journey crisscrossing five states.
Day 1: The Awakening
Day 2: Deep Impressions
Day 3: Music, Montgomery, and More
Day 4: Looking Back, Looking Forward
Day 5: Learning to Remember
Day 6: The Mountaintop
Day 7: Slavery and Beyond
Day 8: Lessons to Bring Home
Day 9: Final Lessons